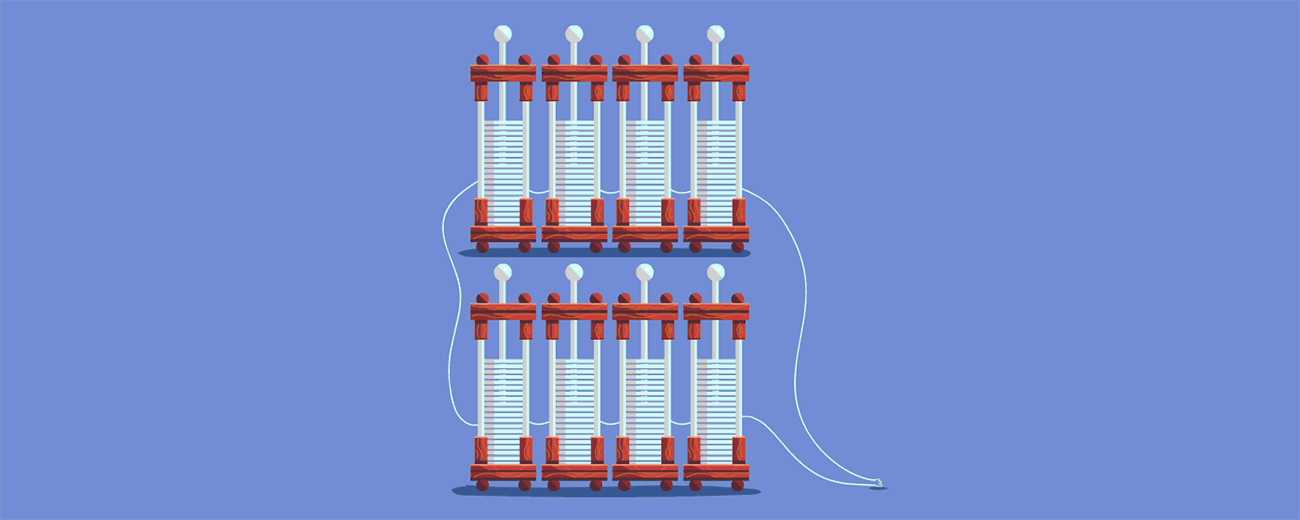
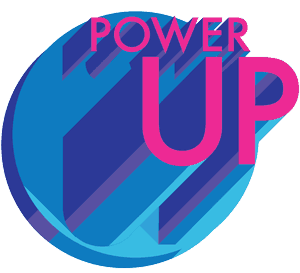
At three minutes past eight on a chilly Tuesday morning in January 1803, George Foster staggered up the stairs to the gallows outside the Old Bailey courthouse in London. The week before he had denied the charges set against him. The judge rejected his plea. Foster was convicted of drowning his wife and daughter, whose waterlogged bodies had been found in early December, in the Paddington Canal. He had refused food and, enfeebled by hunger, had to be helped into the noose. A prayer was said. A cap was pulled over his eyes. Some of his friends stood beneath the gallows, ready to tug on his legs when they dropped, in order to hasten death and limit suffering. The stage fell and Foster was, in the words of The Newgate Calendar, “launched into eternity.”
Eternity was not, however, the end for him. Foster’s body was immediately cut down and carted to a nearby house, where Giovanni Aldini, a 40-year-old Italian physicist, sat waiting–in anticipation of an experiment that he hoped would disprove, in a way, the existence of batteries. In the house, among a small yet influential audience and with a certain amount of dramatic flourish, Aldini inserted one metal rod into Foster’s lifeless mouth and another into his ear. Reports of what happened next would inspire Mary Shelley, who was only 5 years old at the time, to later write Frankenstein, the novel in which a grimly inventive doctor fashions a creature from discarded human body parts.
At first, Foster’s jaws began to quiver. Then the adjoining muscles contorted, opening one of the dead man’s eyes. As electrical current continued to flow through his muscles, Foster’s right hand clenched into a fist, and his legs and thighs began to shake. Some of the bystanders recoiled, believing that he was in the process of being resurrected. Mr. Pass, the beadle of the Royal College of Surgeons (who had allegedly hurried Foster’s trial through in order to produce a fresh body for the experiment), was so alarmed by what he saw that later in the afternoon he died of shock.
While Aldini’s experiment might appear as though it was staged to alarm or entertain, it was, in fact, the latest ploy in a long-running vendetta between two camps of scientists. Aldini’s uncle was the surgeon Luigi Galvani who, two decades earlier, had been watching his assistant dissect a frog when the steel scalpel touched a brass hook holding its leg in place. The leg twitched inexplicably. This set off a chain of experiments, in which Galvani jabbed frogs with different combinations of metal objects to observe the effects. He believed the muscle spasms were caused by “animal energy,” which resided in the muscles themselves, and published his findings in 1791. The reaction, which Galvani’s nephew showed on Foster’s body to such great effect, became known as “galvanising”–and Galvani was hence forth identified as the “frog-dancing master.”
Italian physicist Alessandro Volta didn’t buy it, however. Volta, one of the world’s leading experts in electricity (from whom the volt takes its name), replicated Galvani’s experiments and reached a different conclusion. He believed the frog’s corpse did not contain latent energy, but rather that the chemical-rich carcass was merely acting as a conductor for electricity running between two metals. To disprove the existence of “animal electricity,” in 1799 Volta replaced the frog’s leg with brine-soaked paper, inserting it between a stack of discs of two different metals. This “voltaic pile,” as he named the device, was the progenitor of the modern battery. (Benjamin Franklin had already coined the word itself to refer to a series of Leyden jars, which are able to hold and store a small amount of electrical charge but bear little resemblance to today’s batteries.)
It would be years, however, before the feud between the two camps resolved. At one point, Volta wrote to a friend that his opponents wanted him dead. Even Napoleon Bonaparte got involved, taking part in an experiment with Volta in which he drew sparks from a battery during a series of lectures at the French National Institute in 1800. Galvini’s nephew, Aldini, remained unconvinced, continuing to believe in his uncle’s beloved “animal energy” even after 1802 when the Scottish chemist, William Cruickshank, designed the first electric battery suitable for mass production.
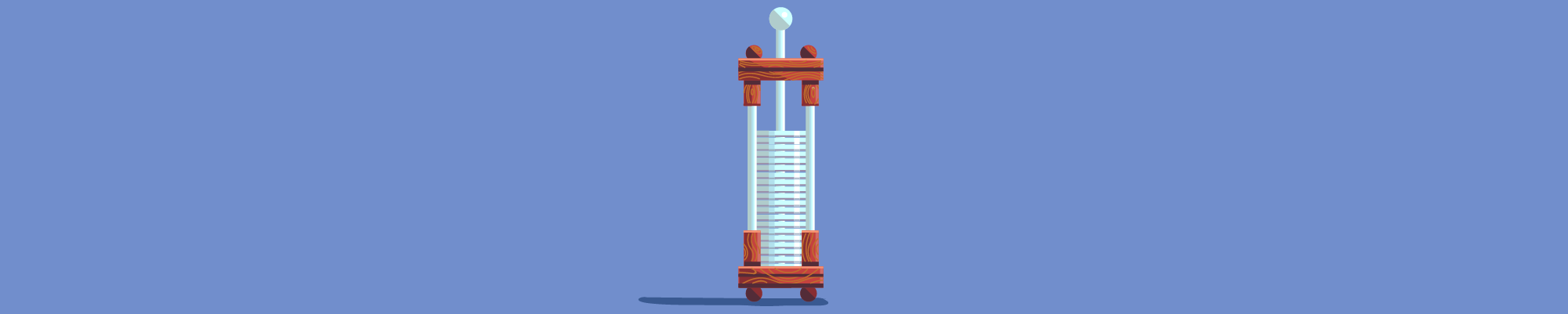
Batteries transformed the world. In the 19th century, before the advent of electrical grids, they were primarily used to power telegraph networks, telephones, radios, and other similar equipment. Today they fuel our wristwatches, save our progress in video games, allow the deaf to hear, keep our hearts beating, and have even sent a man to the moon. In particular, the lithium-ion battery, first proposed by the English chemist M. Stanley Whittingham in the 1970s and eventually made commercially available in 1991 by Sony, changed everything. Today, via laptops and mobile phones, it powers a vast number of the contemporary world’s working and leisure activities.
The science of today’s batteries is almost identical to that of Volta’s pile. New substances such as sulfuric acid (lead-acid battery), potassium hydroxide (nickel-cadmium battery), and lithium (lithium-ion battery) may have replaced the brine-soaked paper, but in each case the chemical performs the same function: acting as an electrolyte to store electrical energy. The chemical produces a reaction when a device (think a light bulb or toy robot) is connected; electrodes create a flow of electrical energy.
If a battery is disposable, it will produce electricity only until there is no more chemical energy left to be transformed into electrical energy. Rechargeable batteries, however, invented in 1859 by the French physician Gaston Planté, allow this process to reverse. Electrical energy from a charger or dynamo is applied to the chemical system, reversing its operation, thereby restoring the battery’s charge.
While lithium-ion batteries have steadily improved over their 25-year history (in 2014, for example, Panasonic created a pin-shaped version that weighs only 0.6 grams), technological advances in mobile phones and laptops have outpaced these improvements. No longer merely an alarm clock, calendar, and telephone, smartphones now double as social media hubs, music players, compasses, video game consoles, TV sets, streaming devices, and almost as many different kinds of things as there are apps available to download–all of which place ever-greater demands on batteries. In one recent case, for example, uninstalling the Facebook mobile phone app reduced battery consumption by up to 15 percent. Where once you could expect to squeeze a few days’ use from a fully charged phone, today many people don’t get through an afternoon without a top-up charge.
“Essentially, we are dealing with the two percent problem,” explained Adam Best, a senior researcher who specializes in battery technologies at CSIRO, Australia’s national science agency. “A common 18650 cell used in laptop battery packs contains only two percent lithium. This does all the work in the cell–the rest of the materials are just host structures, separators, solvents, and the can.” As the amount of lithium in batteries must remain around this level–in order to keep their size to a minimum–batteries have failed to keep up with Moore’s Law, explained Best. Moreover, the materials that comprise the active components of batteries are much the same ones that Sony brought to the market more than 20 years ago.
Essentially, then, researchers have been unable to discover lighter, highly conductive materials to replace lithium, which is the lightest of any solid element. While there have been plenty of potential candidates, in almost every case to date engineers have found that the electrode structure breaks up too quickly, leading to poor cycle life. The technology exists to create super-batteries, but no company has yet found the charmed combination of affordability, lightness, and longevity that would allow the world to move away from its reliance on lithium.
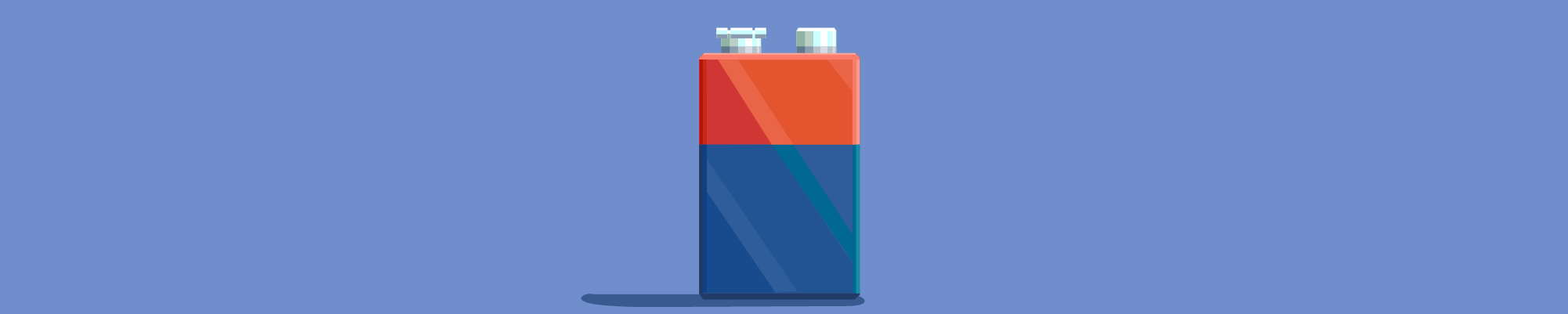
The need to find a new solution is not only related to the increasing demands that humans place on batteries. A 2015 U.S. Geological Survey estimates that the world has enough known lithium reserves for about 365 years of current global production (around a quarter of all extracted lithium is currently used in batteries). But with the advent of electronic vehicles, demand, many speculate, is about to rise precipitously–meaning that estimate may be grossly exaggerated. The trend is already visible. Worldwide production of lithium doubled between 2000 and 2010, and has doubled again since.
Lithium is found in the Earth’s crust, principally in South America where 70 percent of the world’s deposits are located. Prior to the invention of the lithium-ion battery, the metal, which is never found in its purest form in nature, was primarily used as a drug to treat mania. Demand was low. In the early 1990s that all changed, and with increased demand came new pressures on those countries where deposits are found.
The Salar de Uyuni is a bright white lake that covers 4,000 square miles [10,360 square kilometers] of Bolivia. It holds one of the world’s highest concentrations of lithium–around 5.4 million tons of the world’s total 11 million [or 10.8 billion pounds of 22 billion]–resulting in a silvery appearance. Local Indians relate a legend that the Salar was created when the goddess of a nearby volcano pulled her baby from her breast, causing milk and tears to mix and spill, forming the body of water.
The process of mining the lake involves pumping brine to the surface, allowing the water to dry in evaporation pools, then harvesting the minerals that remain. For centuries the indigenous people have harvested salt, wearing wooden masks to protect their eyes from the sun. But researchers believe that mining lithium on a large scale could destroy the Salar’s ecosystem, warning of “mountains of sludge.”
The mining process uses toxic chemicals which require a type of waste treatment that, if mishandled, can contaminate the water. Water depletion as a result of mass-scale mining can reduce resources for local populations, flora, and fauna. The risk of spills, particularly in countries like Bolivia, which have poor roads and infrastructure, increases. More broadly, Bolivia’s relative poverty also makes it a prime target for exploitation. In 1990, the country’s president, Jaime Paz Zamora, agreed to a multi-year contract with the Lithium Corporation of America–one that allowed the company to freely mine lithium, giving Bolivia only eight percent of the profits.
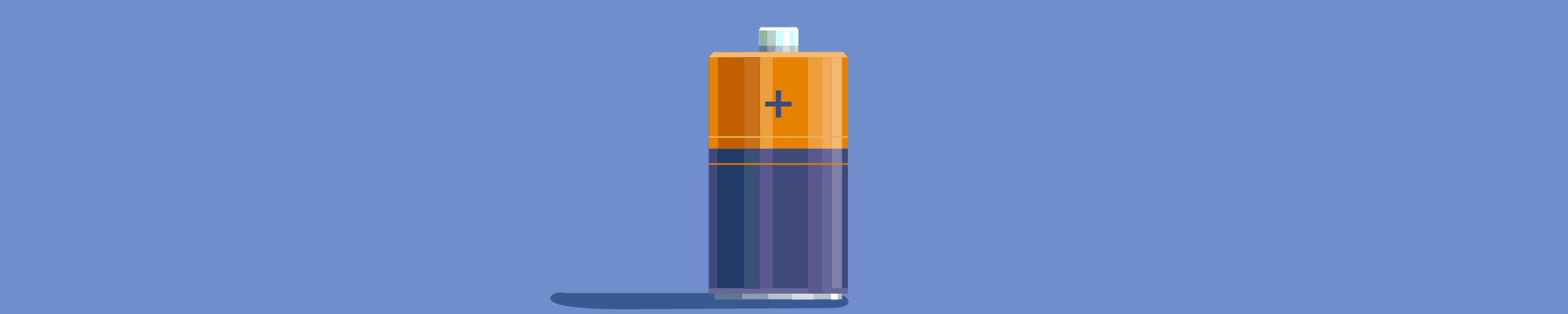
In addition to the problems associated with mining lithium, there’s also the issue of disposing of the batteries it fills. There is, despite research efforts, currently no full-scale recycling mechanism available for lithium-ion batteries. Most, if not all, end up in landfills (only the lithium itself can be recycled)–something of an environmental catastrophe, considering the toxic materials they contain. A 2013 study produced by Abt Associates for the U.S. Environmental Protection Agency found that cobalt and nickel, which are used in these batteries, can have negative impact on human health, leading to respiratory problems, pulmonary, and even neurological effects. A 2012 study, titled “Science for Environment Policy,” published by the European Union states that lithium-ion batteries are a hugely energy-consuming technology, using the equivalent of 1.6 kilograms of oil per kilogram of battery produced. In terms of greenhouse gas emissions, lithium-ion batteries are among the worst offenders with up to 12.5 kilograms of CO2 equivalent emitted per kilogram of battery.
There can also be problems long before lithium-ion batteries reach the landfills. The solvents used in these batteries, such as diethyl carbonate, often cause fires–not least because they must retain 30 percent of their charge in transit. In 2013, for example, lithium batteries set fire to Boeing’s 787 Dreamliner at Boston Logan International Airport. There are already tens of thousands of YouTube videos of so-called hoverboards, last Christmas’s hit gadget, catching fire. “State-of-the-art lithium-ion batteries are unsafe, toxic, and expensive,” said Dr. Kang Xu, a senior research chemist at the U.S. Army Research Laboratory. “They face challenges of large-scale applications such as vehicles and grid-storage. We simply have to resolve these safety, environmental, and cost issues.”
Xu and his colleague Chunsheng Wang from the University of Maryland, however, believe they may have found a solution: a non-flammable, water-based salt solvent that is able to carry lithium ions. The result could be a battery that packs equivalent power to a conventional lithium-ion one but comes with none of its risks. “Our technology aims at absolute safety and non-flammability and already approaches the energy density and cost of lithium-ion batteries,” explained Xu. The project is, however, in its early stages. While Xu claims that progress is steady, the team’s first-generation “aqueous battery” is only a proof-of-concept.
Lithium-sulfur batteries are another alternative battery type looking to come to market. The U.K.-based company Oxis Energy hopes to begin selling this type of battery for electric cars and solar energy storage later this year. Its batteries use sulfur to soak up electrons, offering, in theory, five times the power capacity of traditional lithium-ion batteries. This would, for example, allow an electric vehicle running on a lithium-sulfur battery to travel much farther than one using a lithium-ion battery, or to store far greater quantities of solar energy.
Problematically, though, lithium-sulfur batteries have only a short working life. The chemicals tend to react together, clogging pores in the cathode and causing the sulfur to decompose. Best, whose research is focused on the technology, remains hopeful. “If we can stabilize the lithium metal anode in lithium-sulfur batteries, I can envisage devices with equivalent performance of lithium-ion but in packages potentially two to three times smaller,” he said. “This could be a step change required for lighter electric vehicles, smaller energy storage units for the home, and batteries that last longer in your cell or tablet PC.”
Finally, many believe that lithium-air batteries, which generate electricity by combining lithium with oxygen to form lithium peroxide, could become widespread–although the engineers at the University of Cambridge who are pioneering the technology estimate that it will be a minimum of 10 years before a commercial version is feasible.
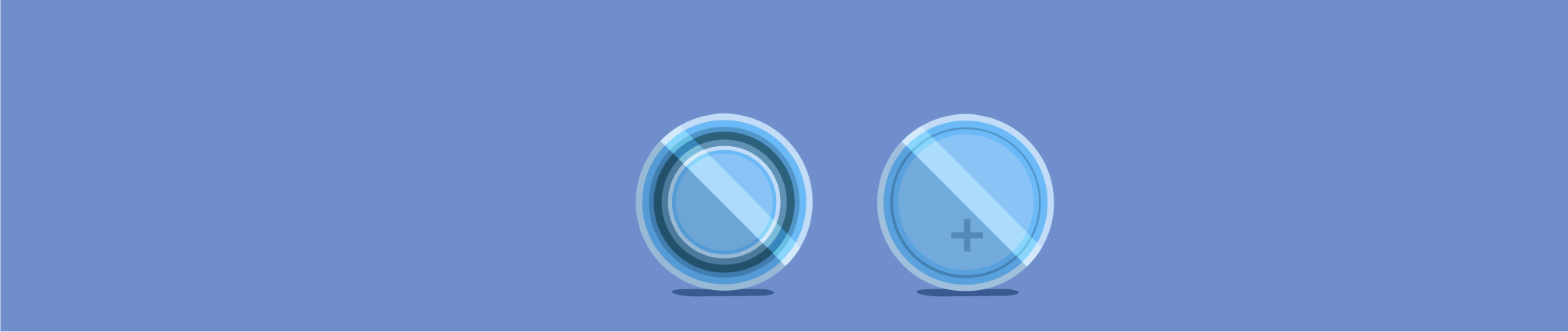
These solutions neglect the problem of lithium-dependence, which could have wider-reaching consequences than its environmental impacts. Much of humanity’s current vision of the future is reliant on batteries. If we fail to find a superior replacement to lithium-ion batteries, the widespread adoption of electric cars and off-grid power solutions could be stymied. And when lithium reserves finally run out, everything could grind to an immovable halt.
To circumvent the problem of lithium supplies, some researchers are attempting to move away from the metal entirely, in particular toward sodium-ion technologies. Sodium’s abundance (it makes up more than 2.6 percent of the Earth’s crust) makes it an attractive, low-cost alternative. These cells can also be completely drained without damaging the active materials, enabling them to be stored and shipped safely, unlike lithium-ion batteries. Best remains unconvinced. “Sodium-ion batteries are even more problematic than lithium ones!” he said. “Sodium metal is more reactive than lithium metal, whilst the batteries themselves are much heavier and have poorer cycling performance.” The reasons for this are elemental: Sodium atoms are simply larger and heavier than those found in lithium.
Every current alternative to lithium has, according to Xu, “certain restrictions and compromises associated.” And, despite the issues that accompany lithium-based batteries, Best doesn’t believe that we necessarily need an alternative. “At the moment, lithium batteries can satisfy nearly all our needs for energy storage.” The problem may be, simply, our capitalistic demands for ever smaller, lighter, cheaper, and more powerful devices.
For Xu, despite rising costs and the threat of scarcity, lithium will likely remain at the core of future battery technologies. “In terms of storing-efficiency, lithium cannot be replaced unless significant compromise is made in terms of energy density. This is simply determined by its position on the periodic table. It is impossible that lithium would become obsolete in batteries.”
There could be one solution, however–a complete step away from Volta’s style of battery. “I think the future will run on super-capacitors, not batteries,” said James Tour, a professor of chemistry and nanoengineering at Rice University. Super-capacitors are different than lithium-ion batteries in that they store energy by means of a static charge, as opposed to an electro-chemical reaction. In this way they eliminate the need for a chemical electrolyte, thereby making lithium obsolete.
“Super-capacitors have a much simpler design and are far easier to make,” said Tour. “Their power output is much higher, which is ideal for accelerating a car, for example, and they charge much quicker than batteries.” Indeed, while the Tesla Model S car has a range of 250 miles or more on a single charge, it could take more than 43 hours to charge the vehicle using a U.S. standard 120V wall socket. A super-capacitor solution would cut that time to more practical levels. “The only drawback is that they cannot store energy as efficiently yet, but that’s improving all the time,” Tour added.
In truth, this isn’t the only drawback. Currently, a super-capacitor able to replace a standard car battery would have to be around 10 times its size. Super-capacitors can be easily damaged if they are overcharged, and they are far more expensive than lithium-ion batteries. For Tour, however, these are problems worth solving. “If we can avoid need for lithium, that is good,” he said. “It would eliminate the need for lithium mines, and the associated costs of shipping the metal.”
The future will, most likely, feature a smorgasbord of different battery types: lithium-ion, lithium-air, sodium-ion, super-capacitors, and perhaps others, too. The choice of battery type will increasingly depend on the needs of the device itself, prioritizing capacity, longevity, lightness, flexibility, or something else entirely. Self-driving city cars might use a different battery-based solution than those vehicles needed for long but sporadic journeys out of town, for example.
As with the 18th-century debate between the proponents of “animal energy” and Volta’s supporters, currently there is no consensus about which understanding will dominate our future. One thing is certain, however, when considering our current energy grid infrastructure. As Best put it: “Batteries are definitely here to stay.”

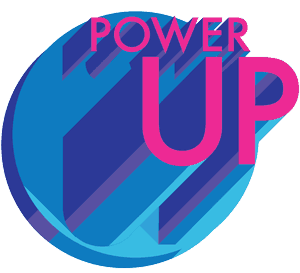
How We Get To Next was a magazine that explored the future of science, technology, and culture from 2014 to 2019. This article is part of our Power Up section, which looks at the future of electricity and energy. Click the logo to read more.