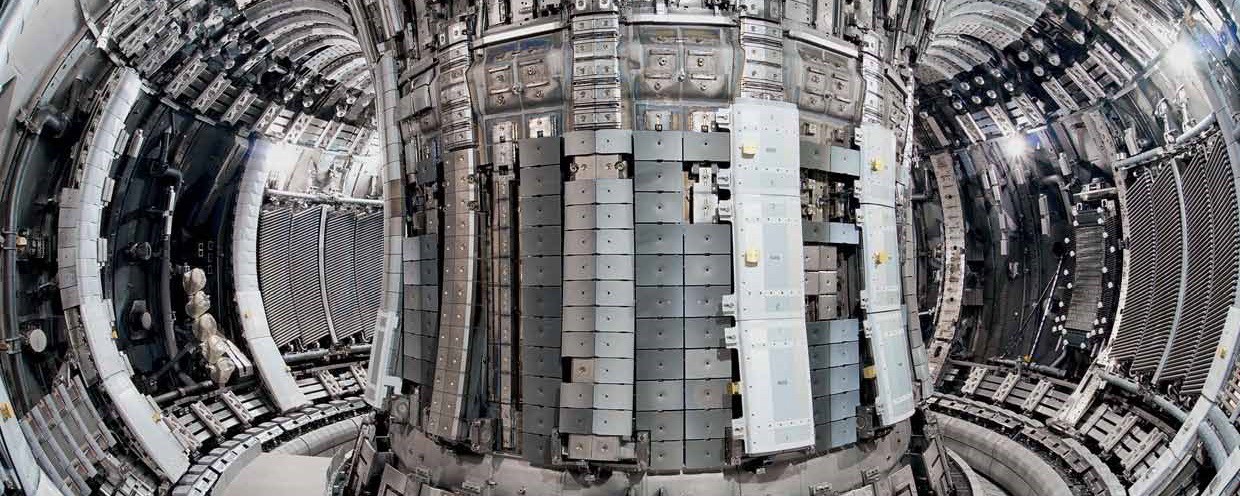
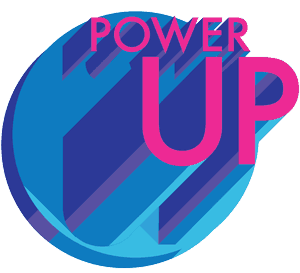
How close are we to stealing starlight for ourselves?
Earlier this month, for the first time ever, German scientists at the Max Planck Institute heated hydrogen gas to 100 million degrees Celsius in a reactor called the W7-X¹, creating a plasma as hot as the core of the Sun for a quarter of a second. Days later, Chinese scientists managed to heat hydrogen to 90 million degrees Celsius for 102 seconds using their reactor, EAST².
These are the latest key moments in the quest to pull off nuclear fusion–the harnessing of energy that comes from smashing atoms together, in just the same way that the Sun burns. (Fission, or what we mean when we refer to the “nuclear bomb” or “nuclear energy,” is the opposite–splitting the atom.)
That said, what happened in Germany and China wasn’t fusion–to achieve that, you’d need to more than double those temperatures–but creating superheated plasma and studying how it behaves is a crucial first step in understanding how we’ll get there. And, in a way, we have managed to create fusion before. The closest thing we have to a nuclear fusion power plant is a reactor called JET³, based at the Culham Centre for Fusion Energy near Oxford, in the United Kingdom. In 1997, it produced 16 megawatts of power for less than a second–that’s about the amount a passenger train generatesâ´. It did, however, take 24 megawatts to power the reactor, so calling the net loss of energy “generation” is innacurate.
That’s just one of the major challenges with nuclear fusion–we know that on paper it’s doable, and that when we scale up a reactor, the power it generates scales faster than the power necessary to start it up. But nuclear fusion is also incredibly difficult to achieve, and requires hugely complex engineering skill and patience.
Right now, an international coalition is at work building the world’s largest-ever reactor in the south of France, called ITERâµ. It should, when it’s completed at some point in the next decade, produce more power than it takes to turn on–and its children will power our civilizations for as long as the stars burn in the sky.
But ITER isn’t the only way. There are other scientists and engineers who look at ITER’s reactor design–it’s called a tokamak–and think they could do things differently, especially as ITER’s costs continue to balloon. (Its budget, now nearly triple what was originally planned, stands at more than $14 billion.) While a tokamak is donut-shaped, there are other models– like the stellarator (that’s what the W7-X uses) or the polywell–which, some claim, could give us workable fusion power generation years sooner than a tokamak, and for a much lower costâ¶.
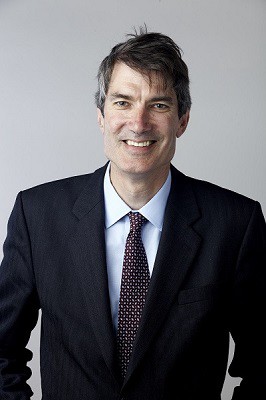
And then there are the engineering challenges yet to be solved. How do you stop the walls of your reactor from turning to mush as their atoms are bombarded by neutrons? Or, even harder, how do you convince short-term-thinking politicians of the importance of funding a decades-long scientific endeavor? And does the world even need fusion if solar energy costs continue to fall?
To answer questions like these, I spoke to Steve Cowley, the CEO of the U.K.’s Atomic Energy Authority, and, since 2008, the director of the Culham Centre for Fusion Energy. Under his watch, the scientists at one of the world’s premiere fusion incubators are doing crucial work on designs for the reactor at ITER; still running JET; and upgrading another reactor, the Mega Ampere Spherical Tokamak (MAST), as the latest in a long history of testing new reactor designs.

So–why fusion?
I think because, if we’re looking toward the end of this century, we’ve got to decarbonize our energy supply. At the moment, 80 percent of the world’s primary energy sources are fossil fuels, and that need is only growing with time despite increased use of renewables. It’s quite remarkable, and, despite concerns about global warming, that is the reality at the moment.
There are ways we can decarbonize rapidly now–for instance, lots of fission power stations and carbon capture and storage, which I would call transitional technologies, because they don’t last a full century, let alone be sustainable for thousands, or even millions, of years. Carbon capture and storage–we will probably use up all the places to keep carbon within a few decades. There’s a limited amount we can do before we use up all the cheap uranium and use up all the world’s nuclear waste repositories. If you take that as a period of time, we’ve probably got 50 to 100 years where the technologies that exist will help us decarbonize. But if you look at the hundred-year timescale, we actually have no recognizable technology that can decarbonize in a sustainable way.
I think there are three options. One is solar–they’ve made marvelous progress with solar, and solar would work if it comes down in cost and we solve the energy storage problem. The second technology that could do it would be advanced forms of fission, fast breeder reactors, etc., but those come with a new set of risks and concerns that at the moment we don’t want to address. And the third technology that can do it and provide millions of years of sustainable, carbon-free, safe energy is fusion. Of the three, fusion is probably the most attractive, in that fusion doesn’t take up much space, it’s safe, it doesn’t produce any waste that is significant, and we’ve got tens of millions of years of the simplest of fusion reactions. And possibly, in millions of years, we’ll be able to do the more difficult fusion reactions, in which case we’ll have more fusion fuel on Earth than is necessary to fuel the world for the lifetime of the planet. There’s going to be no more planet in four billion years anyway, so if you’ve got a source that lasts a few billion years, that’s all we’ll need.
So, fusion looks like the perfect solution, except for the problem that it’s really hard to do. We’ve been trying to do it for 60 years, and we’re capable of a little fusion in JET, and that’s the only machine that’s done any trickling amounts of fusion.
With the recent news from Germany, and then China, it feels like fusion is gaining more public recognition as a realistic prospect–but exactly how realistic is it right now?
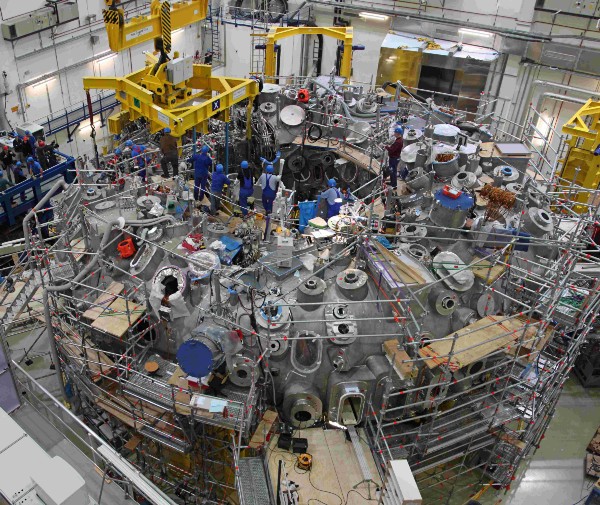
Those are both very good experiments, but they’re not at the scale and power necessary to do fusion. They’re not at the scale and power of JET. JET is the only one that can reach 200 million degrees.
See, JET did 16 million watts of fusion with 24 million watts going in. It’s not exactly how you’d make a power station, no? But ITER is going to produce enough fusion that it keeps itself hot; it’s self-sustaining fusion, and if it does that–it’s an experiment, so I can’t swear on my grandmother that it’s going to do that, all the predictions say it’ll do that–but if it does that, you’ve got to the stage where, scientifically, you can make fusion energy.
Economically, there’s still a ways to go. It’s not cheap. And ITER has been disappointing in that it’s costing a lot more money than we expected it to cost, but energy is a funny thing. It’s a £7 trillion a year market [$10 trillion], and 50 years from now, we’re not quite sure where our energy is going to come from. And without energy, civilization comes to a juddering halt. What percentage of the market should we invest in research? If you’re Sony, you do somewhere between six and eight percent–sometimes as much as 10 percent–of your bottom line is research, because otherwise you will not be in the business 10 years from now. Now, if you take energy, £7 trillion per year, 10 percent of that is £700 billion [$1 trillion]. £700 billion! The total amount of energy research in the world at the moment is about £10 billion to £15 billion per year [$14.3″”21.4 billion]. We’re not taking this problem very seriously.
If you look at modern life, the things that have made life more and more pleasant, in a sense, all use energy. The machines that wash our clothes, the cars that drive us around, everything that’s made modern life more and more pleasant for everybody is energy-intensive.
Factories of the future won’t employ lots of people. Factories of the future will be lots of robots. And those robots–the cost of doing business will be the energy that they use. See, energy sits not just at the core of our society. It’s at the core of our future, too.
And what about this quest most interests you?
The most fun thing I do, still, is physics–to do calculations. I’m a theoretician. I do calculations, on my way home every night. I spend a little time every day talking physics, even though I run a large organization. I like doing physics, but I also like the fact that when I do physics–I don’t on a day-to-day basis think I’m saving the world or anything as grandiose as that–it’s just that this is an incredible problem. How do you make this thing work? We’re close enough to taste it! We’ve actually done some! But we’re still a way from actually making a fusion reactor.
I’m a theoretician, so you ask the question: Theoretically, how good could it be? If we get rid of all the problems, how good could it be? Well, in principle, a fusion reactor could be quite small. It could fit into a largeish room. ITER is enormous, and it’s enormous because inside the fusion reactor the plasma, at 200 million degrees, is bubbling away in turbulence, and that turbulence is letting the heat out. The more we can create less turbulence in there, the smaller we can make the device, and the faster we can get to launching it. And so there’s a clear intellectual drive–you know what it is you have to solve here. You have to drive down the amount of turbulence inside these plasmas, until we can bring the cost and scale of fusion to a level at which private investment starts to work.
I think, if ITER’s successful, and we can demonstrate that and take it and turn it into a reasonably sized reactor, I think money will pour into the industry. If you look around, at the moment there are a whole bunch of private companies looking at fusion.
But what about those private companies–and other national organizations–around the world already working on fusion that use alternative reactor designs?
If you take all of these companies, none of them have really broken through 10 million degrees. And JET does 200 million. So it’s 20 times hotter, and the best of the private companies in terms of performance is a company called Tri Alpha Energy in Irvine, California. They’ve got something like $250-300 million of venture capital, and they’ve achieved about 10 million degrees after 10 years of trying.
The tokamak, it’s a bit of a catch-22, because it’s interesting in that it gives us the best performance, and because it gives the best performance we all tend to bang on about the same thing. And it might be that it hinders us a bit in exploring other configurations that could leapfrog it. It might be. But at the moment, it’s the only one we know will actually take us to fusion.
That’s not to say some bright idea might cut through all the crap and get there faster. When Lockheed Martin had their announcement [in 2014, the company proposed production of a compact reactor with a radical new design within 10 years], they had yet to run a plasma. They had no temperature at all. So there’s an awful lot of hype out there, and I don’t want to downplay the idea that there might be some smart ideas we’ve missed, but it’s got to be based on a sound scientific judgement of whether that’s progress or just hope.
So you’re not worried that by the time ITER is ready to be switched on, a new reactor design will have come along, been built, tested, and put on the market? You feel that the tokamak is still the most prudent avenue of inquiry?
Until we’ve actually managed to make ourselves a first fusion burn, there’s a whole lot of physics that we just don’t know might pop up. ITER’s an experiment, it will teach us things, and if it teaches us that there’s a better way to do it, that would be just terrific. People will say it’s a $15-billion machine, but as I’ve said before, $15 billion is just a drop in the ocean of the problem. And $15 billion over 20 years shared between the seven major partners is a small price to pay for knowing whether fusion is possible.
The Culham Centre’s work is vital to the design of ITER–what kind of research are you focusing on?
Well, we’re involved in this in four ways. The first is that we run JET, and we’re going to put tritium back in JET–the fusion fuel–and break our own record in the next few years. The idea is to break that record of 16 megawatts, and because ITER is not going to be burning a plasma for a decade, it would be nice to have some scientific results right now. That sets us up, because JET is almost exactly the same shape as ITER at its heart but half the size, so everyone learning from JET is learning, getting ready to work on ITER.
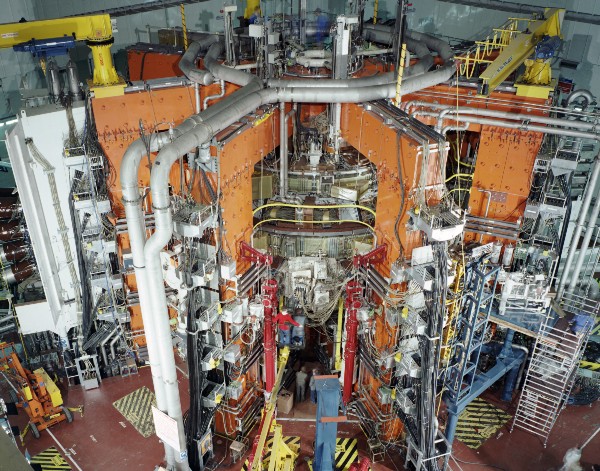
ITER will produce 500 megawatts and we’ve produced 16 megawatts, so it’s a huge difference. Half the size means one-eighth of the body, but the performance scales up, because once it’s powering itself, there’s nonlinear feedback, like lighting a fire. JET is like a fire where you’ve got a gas poker and you’re pointing it at a fire, and some of the wood is burning but it’s not actually caught–it’s not self-sustaining. If you take the poker away, the fire will go out. ITER is like you’ve got it alight; you can add more pieces of wood and it will catch those, too, and you can sustain the reaction.
The second thing we’re doing is building systems for ITER. ITER has to be operated by robots, and we operate JET by robots–they’re remotely controlled rather than being autonomous. An autonomous system would make decisions for itself, but in a nuclear environment, a fusion environment, there’s no need for it to make decisions for itself. For ITER, every system has to have a robotic system that’s maintaining it and controlling it. And we’re doing that with advanced industrial robotics, basically, and we have a new center called RACE–Remote Applications in Challenging Environments–to develop robots that can go into very tricky places. In this case it’s fusion, but we’re also doing it for the nuclear industry, and the oil and gas industry, etc.
The third thing we’re doing is we’re building MAST Upgrade. We’ve shown that by shaping the magnetic field in a different way, and making what we call a spherical curtain, we can reduce the turbulence and make the machine smaller. It’s about pushing the idea that beyond ITER, we’ll go for smaller and cheaper reactors. It’s very difficult to imagine developing fusion if every step costs tens of billions. We want to get to a stage where reactors are of a more manageable size, and MAST has shown that we can get to fusion-type conditions–not quite the performance of JET, because it’s a tiny fraction of the size of JET–but fusion-type conditions with a much smaller machine. That machine goes into commissioning in the summer, and we’ll start operation and getting physics results in 2017. It’s called MAST Upgrade for obvious reasons, but it’s almost a new machine because there’s so much new stuff involved.
The fourth thing we’re doing is developing theoretical models. At the end of the day, just doing fusion is not enough. We’ve got to do fusion at a cost people want to pay for. Part of that is modeling every part of the system. All the physics, all the engineering, everything, we can optimize in the computer, which is a lot cheaper than building many, many devices. That’s the way of modern engineering–a lot of engineering these days is virtual engineering, the way that companies can reduce their development costs and produce better products. We’ve got to do it in fusion because the cost of every step is very, very expensive.
Presumably being able to point to things like new robotics technology makes it easier to get the government to see that there can be a more immediate financial return on your research?
Right. Government is very keen on us playing a role in stimulating industry by doing these very high-tech things, because Culham is one of the U.K.’s greatest assets in terms of science and technology, and fusion is… they’ve always had this problem where fusion is a bit too far away for any politician to care too much about. It’s beyond the political horizon.
Long-term thinking is not a specialty of many governments.
And you can see why. It’s interesting–when I speak in public I get great feedback from people because they care about what happens to their grandchildren. But on a day-to-day basis, government spending on these areas is harder to do, so we have to justify it not just with: “Fusion is the power source of the future.” We have to justify it with: “Look at these contracts we have for robotics and materials.”
We’re doing a lot of research on the nuclear materials of the future. What limits the performance of a lot of nuclear power stations is that the neutrons that come out of fission reactions degrade materials. So the steel and the carbon and things becomes less and less strong, and the reason you close things is because they’ve got to a point where you can no longer be sure they’ve got integrity. And the lifetime of the fuel rods that go with nuclear reactors is dominated by that. You stick them in for a certain period of time, because the cladding, the stuff on the outside, has degraded to a point where you can’t use it in a reactor anymore. If you can improve those materials, all those things get better and cheaper.
So we have to do that with fusion, too, because a fusion reactor will have neutrons. The neutrons that come out of the fusion reactor will rearrange the nuclei in the walls. They will hit the nuclei in the steel in the walls, and that nuclei recoils, and it sort of rearranges all the atoms inside the steel, and if you’re not careful you lose the strength of the steel after a while. And we’ve been developing steels that are very resilient to that–you move the atoms around but they all fall back into place and maintain the integrity of the steel.
It started almost with trial and error, but these days the computer programs that can simulate these processes can allow you to do designer materials. This is the big frontier of material science. It used to be the way you made new materials was cookery–you tried adding a bit of this and a bit of that, and then you tested its strengths and said, “that one’s stronger and that one’s weaker, that one corrodes too fast,” or whatever.
Now what you can do is calculate the properties of materials from the basic interactions of the atoms inside the material. And so you can make a material on the computer and you can optimize it on the computer and then you go and test it, which cuts down the number of steps you have to do in order to get a new material to market.
It typically takes 20 years to get a new material to market, and if we could reduce that time by a factor of two, you’d give your industry a leg up, and everything from turbine blades in jet engines to fusion reactors will benefit from that.
You’ve mentioned several times that fusion research gets a drop in the ocean in funding compared to the problems it could solve. Is it hard to convince people–to convince governments and other bodies–to support your work?
Well, spending the money that we spend on fusion, it’s a drop in the ocean compared to what we need to spend to solve the problem of energy–but if we’ve got no confidence that it will work, it’s still a lot of money. And so you have to make a case based on the science that we’re making progress, and there’s a good possibility that we’ll get there. It’s very hard to do that for government, because they have limited budgets. They have limited understanding of the science, and also we’re competing with voices that are saying, “yeah, fusion might get there, but you know, in the meantime “¦ “
My view is that there isn’t enough being spent on all the energy technologies, and I wish my colleagues in all the energy technologies would say, “hey, you know, the problem is not spending too much here and not enough there; it’s that you’re not spending enough anywhere.” The problem is so existential, we need to step up our game across the board. I’ll be very happy if someone else solves the problem, a problem that my grandchildren will face.
There’s been marvelous progress in areas of solar, and there’s some really brilliant ideas out there. I think that we need the next-step change in solar before we’re really talking about something that’s seriously competitive with fossil fuels. That’s one of the difficulties. Fossil fuels are amazing stuff. When they first found oil, you basically just stuck a pipe in the ground and the oil pumps itself out. You’d have gushers and things, it’s just amazing. That led to this incredibly available, incredibly useful product, and so competing with it means you need a price point into the market that’s incredible. Gas in the United States is just so cheap right now, and it’s difficult to imagine people combating climate change with fossil fuels being this cheap. Almost nothing can compete with that, and when are people going to say “climate change is more important than economics”? Because it’s in your economic benefit to keep burning those fossil fuels.
It feels like you’re arguing that the best long-term mix is fusion plus solar.
I think that’s right, actually. I mean, I don’t think anybody thinks one thing is going to be it–it depends on the country, right? In Britain, wind can do quite a lot. It’s a 30 percent kind of thing, which is good, because solar’s going to be much harder in Britain than in other countries, and we’re lucky to be blessed with a mighty wind.
But on the other side, you’d like something that’s on all the time, and fusion’s perfect for that. I have this line I tout around which is that it’s the perfect form of energy except for one thing–it’s really hard to do. And when we know how to do it, we’ll never not know after that point.
There will probably be a few ideas we haven’t yet had, and I’m concerned–and remember, I run one of the biggest labs in the business–I am concerned about the cheap, innovative side to the fusion program. When you’re building something as big as ITER, there’s a tendency to coalesce around one set of ideas. I’m very happy to see people out there touting new ideas. But some of them–at the same time, you should be very critical, because that’s the nature of science. You can say, “well, you know, that won’t work because x, y, and z.” You shouldn’t be criticized for saying that, because that’s how we make progress.
You have ideas, but you can be enormously critical. We’ve tried a lot of things in fusion–60 years and many, many ideas have been tried. The tokamak just works the first time, but it might be the hot air balloon. It was the first thing to fly, but it wasn’t the answer.

Footnotes
- This stands for Wendelstein 7-X–it’s based at the Max Planck Institute in Greifswald. Chancellor Angela Merkel, herself a scientist before a politician, flicked the switch to turn it on.
- Stands for Experimental Advanced Superconducting Tokamak–it’s based in Hefei, and is one of the most important test sites for ITER (of which, more on below).
- Or the Joint European Torus, to give it its full name.
- Specifically, it’s a little more than what a 20-car Eurostar train draws from the power rails as it runs beneath the English Channel.
- “ITER” isn’t officially an acronym–it’s Latin for “the way”. It was originally meant to stand for “International Thermonuclear Experimental Reactor”, but that was dropped from the organization’s list of official acronyms because of the unpopular connotations of the word “thermonuclear”.
- The tokamak’s doughnut shape is as close to a “standard” design there is when it comes to fusion; the stellerator, by comparison, looks like one made by a child, twisted up into a wavy structure. They work on similar principles–with a hot plasma held in place, away from the chamber walls, by magnets–but the stellerator only uses magnets, whereas the tokamak uses a powerful current passed through the plasma as well. Tokamaks are easier to design and build, but harder to run; stellerators are, theoretically, the opposite. Polywells hold the plasma in place in a single central field, without moving; it makes them much harder to control, and to get energy out of, but they (and other designs derived from them) should be much smaller than other reactor designs, which has made them a subject of particular interest for the U.S. Navy and private contractors who see them as ideal for powering submarines and warships.

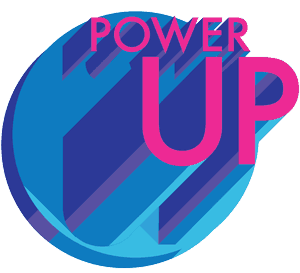
How We Get To Next was a magazine that explored the future of science, technology, and culture from 2014 to 2019. This article is part of our Power Up section, which looks at the future of electricity and energy. Click the logo to read more.