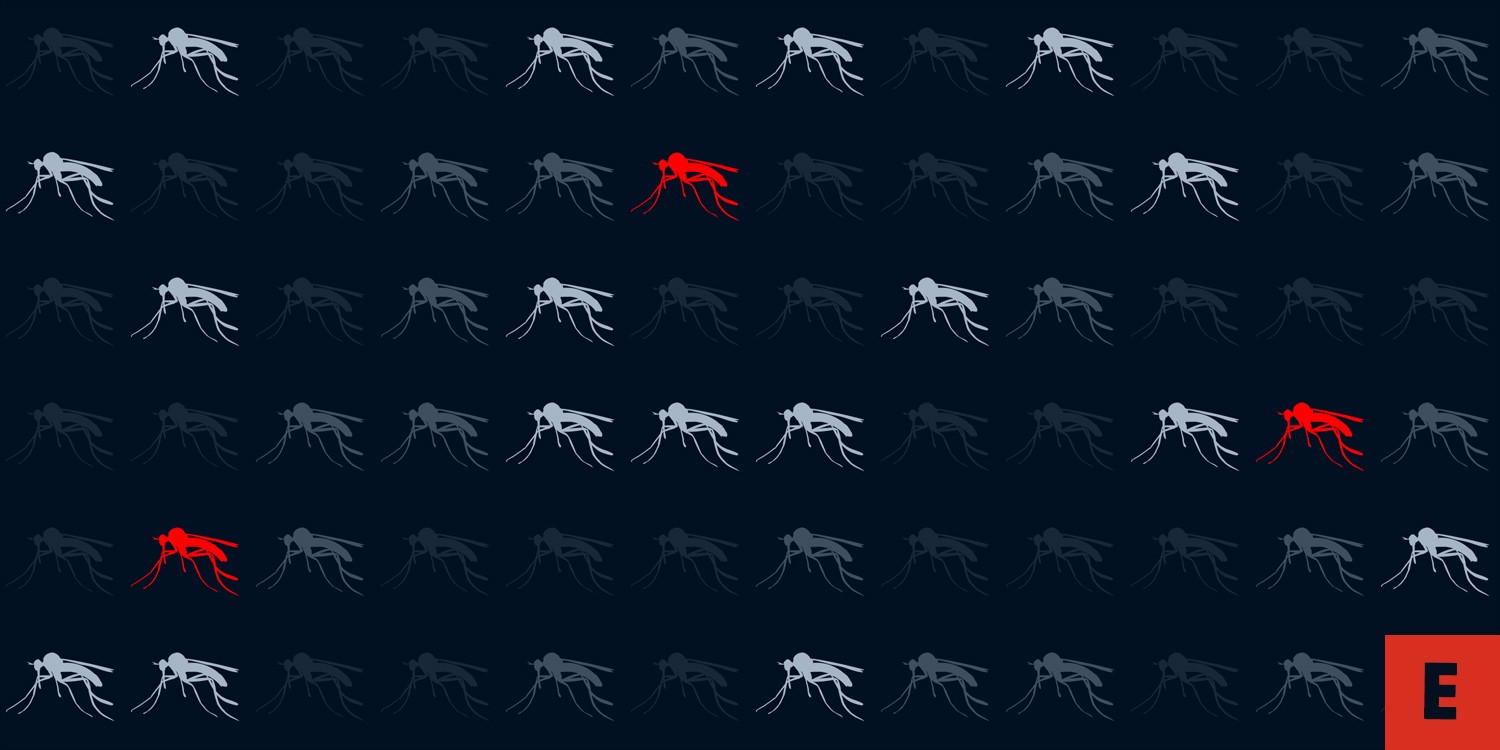
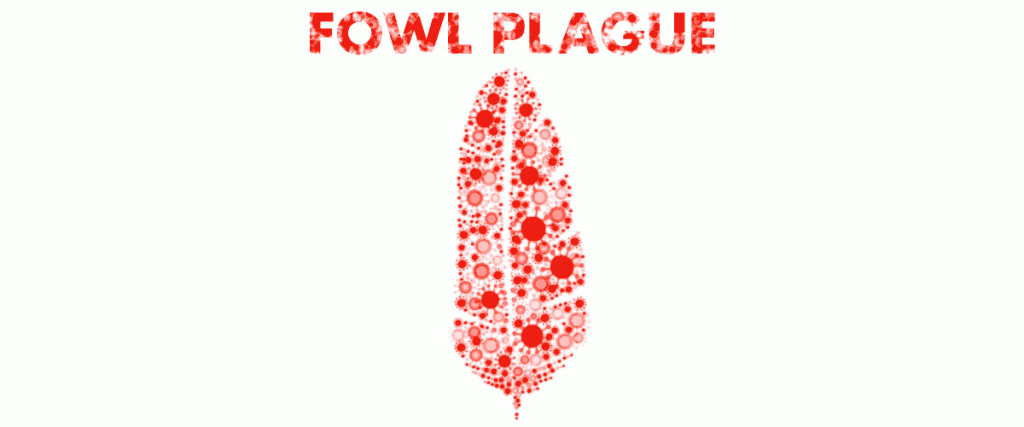
Read the next installment: “Airborne: We’re five mutations from a major pandemic”
Read the previous installment: “Zoonosis: How animal diseases become human diseases“

It’s almost midnight when the mosquito slips into the bedroom through an open window. Lured by smell of breath and sweat, she lands on a portion of exposed skin just above the victim’s thigh. The mosquito then begins to feed, arching her body forward to acquire the blood meal that will sustain the development of her eggs. As she does so, hundreds of protozoan parasites pass through her proboscis and into the victim’s bloodstream, where they travel to the victim’s liver.
There they will reproduce over and over again, until the liver cells burst and spray thousands of new parasites into the bloodstream. Those parasites will reproduce again in red blood cells, and the cycle continues. For the victim, who is now suffering from full-blown malaria, a cycle of chills, fever and headaches will come and go every two to three days, following the life cycle of the parasites. In each cycle, more red blood cells are destroyed and less oxygen, glucose, and iron reach the vital organs. If not promptly treated, the effects progress to severe illness and often death.
According to the World Health Organization, there were 212 million new cases of malaria worldwide in 2015, 90 percent of which occurred in Africa. It’s just one example of many diseases, including chikungunya, dengue fever, and Zika virus, that are spread by mosquitoes. While sucking blood from humans and other large mammals to provide nutrients for their eggs, the female mosquitoes–the only ones that bite–unwittingly ingest and expel harmful pathogens. Tiny, fast-breeding, and with bites often only noticed long after the fact, mosquitoes remain one of the world’s most efficient vectors of disease. And climate change is only increasing their effects.

Historically, the closest thing to a permanent solution to the spread of mosquito-borne diseases lies in “vector control.” That means denying mosquitoes places to breed by draining at-risk areas of standing water, distributing mosquito nets for bedding and windows, and the judicious use of pesticides.
While vector control methods are widely considered to have successfully reduced the number of malaria cases worldwide, the disease remains stubbornly persistent in specific hotspots across Africa, Southeast Asia and the eastern Mediterranean. According to Hugh Sturrock, an epidemiologist at the University of California, San Francisco, recent advances in predictive analytics–the process by which scientists can use data to map the possibility of future outbreaks–could help break the stranglehold the disease has on these areas.
Sturrock heads up Project DiSARM, a system that allows malaria elimination programs to better predict when and where the disease will emerge. “As you get towards the end of an elimination effort–bringing the malaria right down to manageable levels–you end up with these pockets of transmission,” he explains. “Understanding where these final pockets are becomes really important. But to do that, you really need to collect, process and analyze spatial data. And that’s just capacity that these programs often don’t have.”
Elimination projects often try to solve this problem by sending data on outbreaks to outside experts. “That gets analyzed for them, and then some reports or maps are given back to them, which is fine. And you end up with good maps, but it’s not really, in my mind, a sustainable way forward.”
The aim of Project DiSARM is to give the power of prediction back to elimination teams on the ground through the creation of a single platform able to analyze data on elevation, rainfall, temperature, vegetation, and historical outbreaks. For most of its existence, that’s meant producing risk maps of infected areas. Unlike other programs, however, DiSARM uses a targeting module that allows the user to prioritize certain areas for spraying. In the past, such analysis often required help from labs hundreds or thousands of miles from the affected area. Now the process has been simplified: elimination teams can just pick the areas themselves.
“Last year we used a very early version of DiSARM to help [a] Swazi program decide where to spray with insecticide,” explains Sturrock in one of the first practical demonstrations of the platform. It was a dry run–the platform succeeded in pointing out which areas the team should consider spraying–but since then its developers have built in new features that will allow elimination teams to update the risk maps on a day-to-day basis.
“We’re implementing that this year in Swaziland and Zimbabwe,” says Sturrock, with additional trials in Namibia and Botswana. “We’ll have a much better sense of our success with the platform by then.”
With the rapid uptick in smartphone use across Africa and South Asia, the ability to ask local residents the right questions–whether they were bitten by mosquitoes the previous night, if they sleep under a net, or even if it rained recently–becomes much, much easier. Mobile devices could also play a pivotal role in revolutionizing the identification of mosquito species, with research already underway in pairing machine learning with image recognition of individual insects. One group at Stanford University is even experimenting with using acoustic signals picked up by smartphones to identify individual species.
That’s the dream, anyway. For now, Sturrock and his team are focusing on expediting the nuts and bolts of malarial epidemiology: finding the places where mosquitoes breed, and spraying nearby homes and areas of standing water with insecticide. “Countries at the moment are keen to find better ways to record what they do, because spraying is a really expensive activity,” he says. “If they can make it more efficient, and make sure that they’re achieving the targets that they set themselves, that has tremendous value.”

Using data to track down the places where mosquitoes breed is one approach to tackling malaria. But an alliance of entomologists and molecular biologists has been looking at another method that targets the mosquitoes directly: genetic engineering. By tweaking the ability of certain mosquitoes to breed, some biologists hope to drastically reduce their numbers in a targeted way.
“You need to look at the applications of these new technologies in light of the previous, so-called “‘conventional’ vector control approaches,” explains Tony Nolan, a molecular biologist at Imperial College London. Nolan is one of the leads on Target Malaria, a nonprofit research organization experimenting with gene drives to eradicate local populations of Anopheles gambiae in sub-Saharan Africa, thereby helping to thwart the spread of malaria. Unlike the use of pesticides and habitat control, this method promises to affect only that particular insect. “Even in Africa, there’s about 850 mosquito species,” he says. “And this would be targeting only specifically those ones that transmit the most deadly form of malaria to the most number of people.”
The technology works by artificially manipulating genetic inheritance. Normally there are two versions of any given gene present in a living organism. The probability of any of these genes being passed onto future offspring is usually 50 percent, which is how select features perpetuate themselves through the generations. Certain genes, however–so-called “selfish genetic elements”–make extra copies of themselves, and therefore have a much greater chance of being passed down to the next generation. By splicing new “selfish” genetic elements that promote infertility into mosquito DNA, biologists hope that the insects will go extinct.
Gene drives are not the first attempt by scientists to limit mosquito populations through genetic manipulation. A blunter approach, known as Sterile Insect Technique (SIT), was developed in the 1950s along the same principle, albeit using radiation to sterilize the captive mosquitoes. SIT was first used to successfully eradicate populations of the New World screwworm, a fly with a nasty habit of laying its eggs in human scalps. The technique has since been brutally effective in wiping out insect populations in around a dozen locations, including the melon fly in Okinawa, the tsetse fly in Zanzibar, and two species of moth in British Columbia and South Africa, respectively.
The technique has its limitations, however. For it to work, local populations have to have already been suppressed by a combination of other methods, like mass spraying and habitat destruction. Even if this is achieved, irradiating the insects can reduce the natural ability of individual males to mate. Mosquitoes altered using gene drive techniques, on the other hand, do not suffer these side-effects.

It was in 2003 that Austin Burt first outlined the possibility of engineering new features into–or out of–species of mosquito. Burt, an evolutionary biologist at Imperial College, predicted that by splicing a selfish genetic element called an endonuclease into their DNA that would encourage infertility in male mosquitoes, scientists could force the eradication of a population “in fewer than 20 generations.” The technology of the day did not allow Burt to pursue anything even close to that goal. But with the recent invention of CRISPR/Cas9, a genetic tool that allows scientists to cut into DNA and edit gene sequences, this method of population control has now become a real possibility.
There have been challenges. “As with anything that tries to suppress [a] population, there can be selection of resistance,” says Nolan. “Gene drives are no different. There are ways that you can try and make resistance less likely, by targeting multiple [DNA] sites, for example.” It’s a process the molecular biologist compares to combination therapy with antibiotics, where multiple drugs are used at once. If a strain of bacteria is resistant to one drug, then it’ll be killed by the other, and that resistance won’t be able to spread. Similarly, the alteration of a single region in the genome could potentially be thwarted by one naturally-occurring genetic mutation. The chances of mutations overcoming several such alterations is, in the same way, much smaller.
Although Target Malaria’s work is still largely confined to the laboratory, Nolan is confident that it will eventually be introduced to sites on the ground. “We’ve proven [the] principle for certain aspects of this technology in the laboratory,” he says. “But there is a long downstream process whereby we need to show that [gene drives] work over sufficient timescales, at sufficient scaling, [and] that they’re safe.”
The next step is demonstrating the efficacy of the technology to communities in Burkina Faso, Uganda, and Mali, where Target Malaria intends to deploy the technology. In 2012, the organization began training local scientists, fitting out new laboratories and educating communities about how malaria spreads (the belief that the disease is caused by eating greasy food remains persistent in parts of Burkina Faso). Although the necessary infrastructure is being constructed, the organization remains hesitant to explain gene drives in full until the technology is perfected.
“It’s true that there’s been nothing like this in terms of genetic control in the African context, or in any context, actually,” says Nolan. “We’re in the early stages of developing that conversation, but any deployment is a long way away and won’t happen without local approval and local consultation.”

Local approval is something that scientists from a company called Oxitec failed to get from the residents of Key Haven, Florida late last year. Oxitec had arrived at a trying time for health authorities across the region: an outbreak of Zika virus, a mosquito-borne disease that causes microcephaly in newborns, was gripping Brazil and neighboring countries in South America. People from these areas frequently travel to and from Florida, and many of Key Haven’s residents feared that the virus could make its way north in this process. Meanwhile, Aedes aegypti, a species of mosquito that carries Zika and whose range extends as far north as the Great Lakes, seemed to be acquiring a worrying resistance to major pesticides.
Oxitec offered a solution. The for-profit biotech firm had pinpointed Key Haven as the ideal testing site for its genetically-modified mosquitoes–all of the offspring of its insects were designed to die before birth–prior to gaining approval for a wider rollout from the U.S. Food and Drug Administration. Oxitec claimed that a similar release of its modified mosquitoes in Brazil saw local populations of Aedes aegypti plunge by 82 percent. Furthermore, it was safe: the firm’s scientists invited skeptics who were concerned that its releases would lead to more disease-carrying mosquitoes to stick their hands into cages full of GM-modified males to see if they would bite. But local residents were still concerned about the ecological impact the trial could have, and so it was put to a vote.
When the results were announced in November, the verdict from the citizenry of Key Haven was a resounding “‘no.’ The referendum revived age-old fears about the dangers of genetic modification, and it dredged up lingering concerns about Oxitec’s research practices, most notably during field tests the firm had carried out in the nearby Cayman Islands in 2009. A review by scientists from the Max Planck Institute for Evolutionary Biology published in 2012 criticized Oxitec for a lack of transparency in the run-up to and during the trials; the few documents that were published lacked key information and details of scientific methodology.
“It was done very secretly,” says Dr. Christophe Boëte, a researcher at the Institut des Sciences de l’Evolution in Montpellier, France, of the Cayman Islands trials (another peer-reviewed evaluation of the tests was subsequently published in the journal Nature Biotechnology). “Other trials have [also] been done in Malaysia despite the opposition of the local population.”
Nor has gene drives’ impact on local ecosystems been fully explored, Boëte says. Scientists believe an individual species of invasive mosquito could be bred out of existence without damaging local food chains, but there is no way of knowing for certain. “The role of the mosquito in food chains is not that well-measured,” Boëte explains. “You might also have another invasive species of mosquito arriving to take its place.”
Then there are deeper ethical issues over who could ultimately get their hands on gene drives, and to what ends. The eradication of mosquito populations to thwart the spread of malaria might be seen as a noble goal, but Boëte sees an ominous gap in existing regulations. Although the Cartagena Protocol on Biosafety establishes international ground rules in this area, the United States–where a great deal of gene drive research is conducted–cannot sign on to the agreement because the U.S. Senate has not ratified the Convention on Biological Diversity (the United States is the only country in the world that has failed to do so). If proper considerations are not paid towards keeping technologies like CRISPR Cas/9 in the right hands, Boëte says, the consequences could prove dire.
“I think the risk could be strong modification of the ecosystem in an unexpected manner,” he warns. “Obviously you could have the impact you want on your target species, but you might end up having unexpected consequences in the wrong attempt”¦ It’s something that is not taken into account. Business models are not always concerned by the longer-term consequences.”

There is one method of mosquito control that Boëte predicts will, in the long run, prove to be safer, cheaper, and more effective. In 1971 researchers discovered that male mosquitoes infected with Wolbachia, a type of bacteria estimated to infect 52 percent of all arthropod species, will inadvertently destroy the eggs of uninfected females after mating with them. Recent releases of Wolbachia-infected mosquitoes raised in the lab have resulted in marked decreases in local populations. Strains of the bacteria capable of blocking the transmission of dengue fever, yellow fever, chikungunya, and the Zika virus also exist.
Not only is Wolbachia cheaper than a gene drive, but extensive tests proving the efficacy of the method have already been carried out in Australia. “It’s kind of seductive,” says Boëte. “It’s probably more acceptable, and more accepted, to have Wolbachia-infected mosquitoes.” Regarded as a “biopesticide” under U.S. law, it’s “also much cheaper than the Oxitec approach, because you don’t need to have many, many releases.”
Work on introducing Wolbachia-infected mosquitoes into the wild has already begun. In October 2016, the Australian nonprofit Eliminate Dengue announced that it had received $18 million from the U.S. government to begin releases in Rio de Janeiro and Medellin, Colombia over the next two years. This was followed in July 2017 by the phased release of over 20 million sterilized mosquitoes across Fresno County, California, by Verily Life Sciences, a subsidiary of search engine Google’s parent company Alphabet. Back in the Florida Keys, a similar trial is also underway, under the management of MosquitoMate, a privately-held company based in Kentucky.
Despite the current hype, it is by no means certain that Wolbachia is the holy grail in the quest to eliminate the transmission of malaria, dengue, or Zika. Scientists are not entirely sure why the bacteria prevents the further spread of these diseases in mosquitoes, and the releases themselves are still just attempts at a wider proof of concept. According to Scott O’Neill, Eliminate Dengue’s program leader, a more nuanced understanding of Wolbachia’s effect on disease transmission will come when the results of an ongoing randomized controlled trial in Indonesia are announced. “That will provide the gold-standard evidence that epidemiologists would like to see,” he told The Atlantic last October.
If that evidence points to success, Wolbachia may prove to be a mutually beneficial development for both humans and mosquitoes: blocking disease transmission will end the mass extermination campaigns that typically accompany an outbreak of malaria or Zika. If not, then the pace of research into gene drive technology could mean that the questions we ask ourselves about the extent and reach of vector control become much, much broader.

Read the next installment: “Airborne: We’re five mutations from a major pandemic”
Read the previous installment: “Zoonosis: How animal diseases become human diseases“
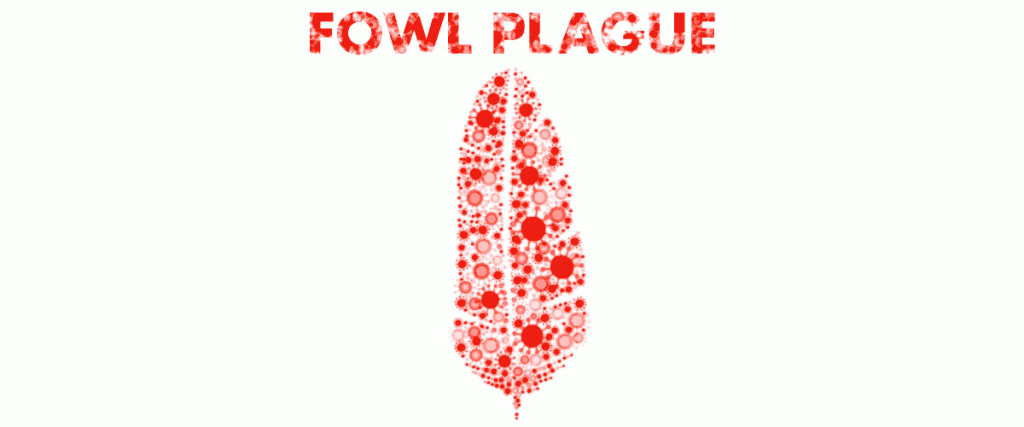
How We Get To Next was a magazine that explored the future of science, technology, and culture from 2014 to 2019. Fowl Plague is a five-part series that explores the history of deadly global pandemics–and asks whether we’re ready to respond to the next one.