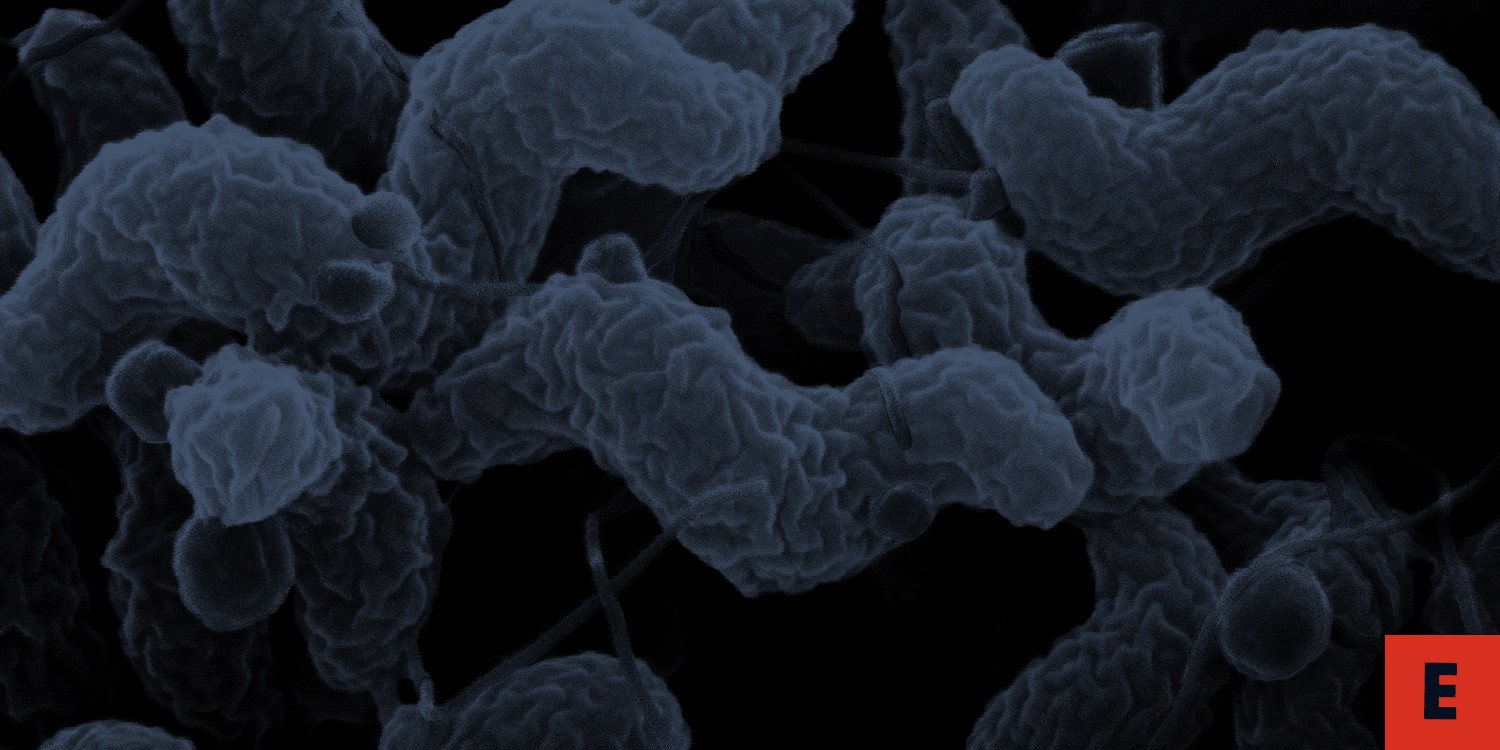
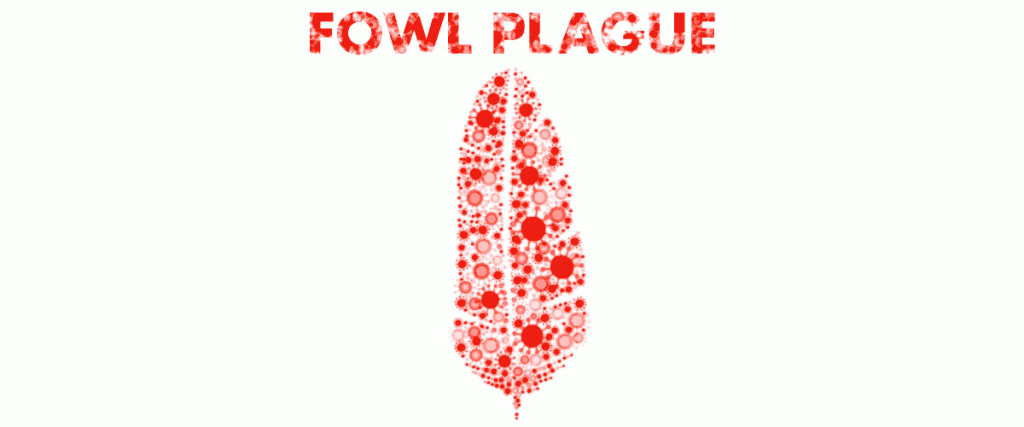
Read the next installment: “The Machine and the Mosquito“
Read the previous installment: “Outbreak: Our next global pandemic“

In 1998, pig farmers in Malaysia suddenly started falling ill and doctors didn’t know why. Within days of their first symptoms–headaches and fever–many of the farmers were hospitalized as their brains swelled. They suffered seizures or fell into comas. About half of those infected died.
More and more new cases appeared, but nobody knew where they were coming from. The Malaysian government blamed the disease on mosquitoes, telling people that everything was under control because they were spraying insecticide. But then one persistent researcher, Dr. Kaw Bing Chua, tested some samples at the Centers for Disease Control and Prevention in Colorado.
Chua found that the disease–which had been named Nipah after the town it emerged in–was a new member of a family called “paramyxoviridae,” which also includes measles. These viruses come from livestock, not mosquitoes, and often infect the lungs–so they can spread through the air. He immediately phoned the Malaysian government and told them the news.
The government listened–and organized the country’s largest ever animal culling program. More than a million pigs were herded into pits and shot. In the months that followed, nearly 300 people fell ill with Nipah; around 40 percent of cases were fatal and another 20 percent of patients suffered long-term neurological effects. But by June the following year the outbreak was over: Nipah had been successfully contained.

Nipah virus was a classic example of a “zoonosis”: a disease being transmitted from animals to humans, or vice versa. Zoonotic disease includes many of our most formidable foes: Ebola, malaria, bird flu, and severe acute respiratory syndrome (SARS), to name just a few.
Another common example is food poisoning, which is often caused by bacteria in the genus Campylobacter. The bacterium usually infects poultry, but the birds display no clinical symptoms. Basic food hygiene practices and thorough cooking are enough to kill the bacterium, but still one in ten people fall ill with a Campylobacter infection each year.
There are relatively few examples of communicable diseases with absolutely no history of zoonosis, but Poliovirus–which causes the debilitating disease polio in about one in four of people infected with it–is one pathogen that is truly unique to humans.
Zoonotic diseases can be transmitted any time there is contact between humans and wildlife. Tending livestock, veterinary work, hunting, and eating wild animals are all common causes. Zoonotic pathogens can be bacterial, viral, or fungal, and DNA evidence suggest that they represent a startling 70 percent of all human diseases.
But a pathogen is not necessarily harmful to all animals it infects. Many species are able to act as “reservoir hosts”–carrying the infection but displaying no symptoms. This can make it difficult to spot new zoonotic diseases before they spread.
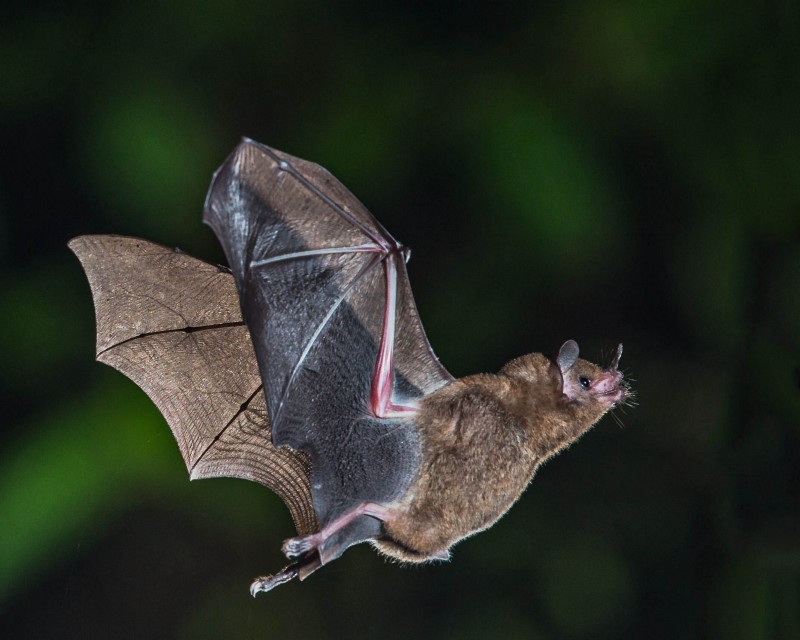
Bats, in particular, have been found to harbor a disproportionate number of emerging zoonoses. SARS, Ebola and even some Nipah outbreaks have been linked to human contact with a type of fruit bat called flying foxes. We don’t know precisely what makes bats so ideally suited to act as reservoir hosts, but their relatively long lifespan, tendency to live in groups, and ability to fly long distances all help. Fruit-eating bats are also extremely common, occurring pretty much everywhere in the world that there is fruit.
Adapting to the immune defenses of a new host species is no mean feat for any pathogen. To most microorganisms, our bodies are as inhospitable as the Moon. The vast majority of diseases in other animals are likely to stay there.
Sometimes a single strain of a zoonotic disease will evolve to infect many different host species. In other cases, adapting to a new host results in an entirely new strain, specific to the new host species. Take human immunodeficiency virus (HIV), for example–new HIV-1 and HIV-2 strains are thought to have evolved from simian immunodeficiency viruses (SIVs) in the first half of the 20th century.
But even after a jump from humans to animals has occurred, the path to a pandemic is not straightforward–HIV-1 M didn’t to reach pandemic levels for another six decades, when growing urban populations and increased promiscuity created the perfect conditions for the disease to spread. Since 1981 this strain has infected 60 million people and caused at least 25 million deaths.

Although animal diseases have probably always infected humans, increased urbanization and international travel have facilitated a boom in zoonoses. Between 1940 and 2004, animal-borne infections represented 60 percent of all emerging infectious diseases. China, which researchers believe to have been the origin of the Black Death in the 1300s, has been described as a “cradle of zoonosis” in modern times, because of its combined profile of rapid urbanization, globalization, and income growth. It was the birthplace of H5N1 avian influenza in the 1990s and SARS in the 2000s.
China isn’t the only source of zoonoses. The recent Ebola pandemic caused devastation across Africa and panic around the world. Like HIV, Ebola is not a new disease–it has existed at relatively low levels since the 1970s. Small outbreaks periodically erupted in humans and wildlife, but it wasn’t until 2013 that the disease reached pandemic levels, killing over 11,000 people worldwide.
Research interest in zoonotic diseases has spiked in recent decades, but even accounting for this extra effort, the number of emerging infectious diseases has increased steadily since 1940, with the majority originating in animals. This seems to be linked to a growing human population that is wealthier, travels more, and is living in increasingly dense urban areas, offering plenty of opportunities for diseases to spread.
An analysis of zoonotic diseases affecting over 2,800 species of mammal found that zoonoses are more likely to spill over into humans from species whose range overlaps with either those of other mammals, or with urban environments. The viruses most likely to make the jump to humans were those that already infect many species, are transmitted by arthropods such as mosquitoes and ticks, and those that possess certain cellular traits.
Climate change is also lending a helping hand. In 2016, researchers led by Kate Jones and David Redding at University College London developed a computer model to predict future outbreaks of Lassa fever in Africa. Lassa, which currently infects up to a million people a year in sub-Saharan Africa, causes a hemorrhagic fever that can be fatal.
The model predicted that cases of Lassa will double by 2070. The increase will largely be driven by climate change, which will expand the suitable habitat for the species of rat that spreads the disease, along with growing human populations and urbanization. The team said that their model could also be applied to predicting future outbreaks of Ebola and Zika virus.
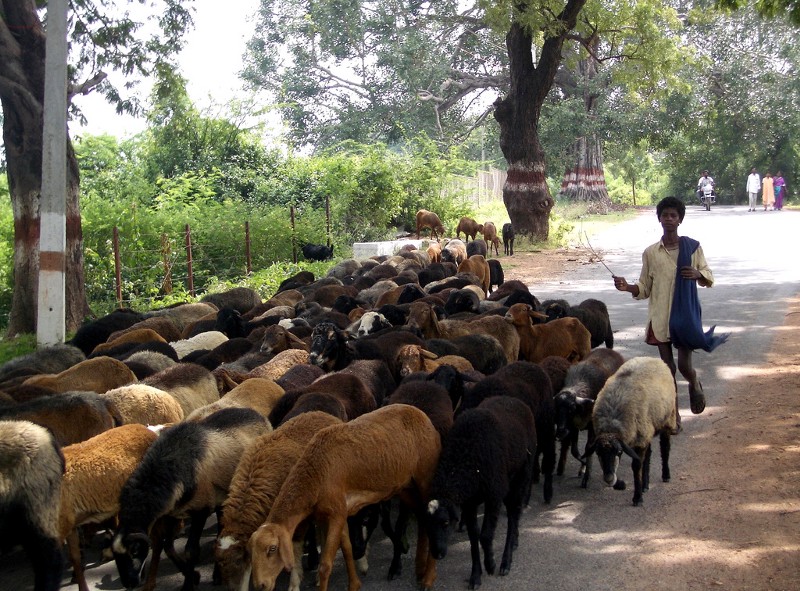

We can’t stop diseases from making the jump from animals to humans. But by predicting which diseases represent the highest risk, we can take steps to reduce contact with host animals, vaccinate humans and animals against infection, and monitor new outbreaks to prevent them developing into pandemics.
Databases tracking human outbreaks of known pathogens can offer an early-warning system for pandemics, and some novel surveillance systems have recently been developed to monitor the levels of disease in wild animal populations. One new technique uses blood-sucking flies as flying syringes, collecting samples of blood from wildlife that can later be tested for zoonotic diseases by scientists.
Vaccination can also defend us against known zoonotic diseases, but research and development efforts tend to be skewed towards pathogens affecting the developed world, missing major hotspots of zoonoses in developing regions. And while vaccination programs may be effective for livestock, they are more challenging to administer to wild animals.
Those wild animals present an limitless pool of potential new diseases that could threaten humans. Targeted monitoring and containment, combined with preventative measures such as education programs to reduce bush hunting, or improved farm designs that minimize contact between livestock and wild animals, may be our best hope to minimize the risk of pandemic.
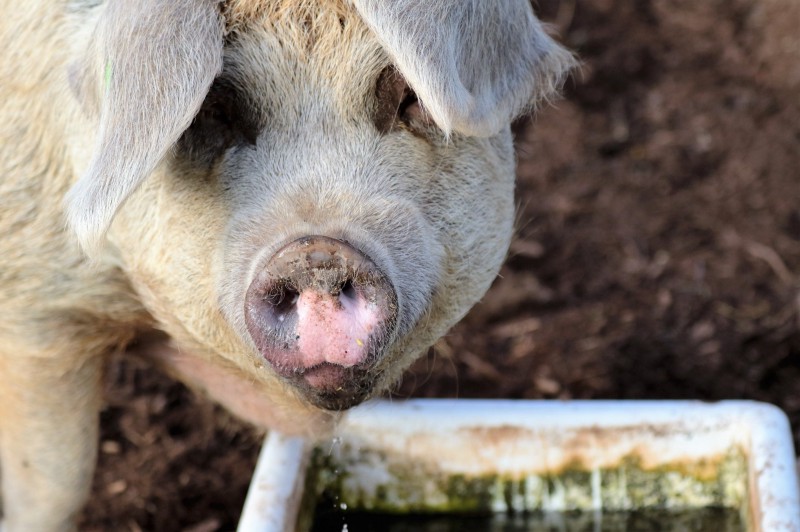

As the 1999 Nipah virus outbreak came to an end, both Malaysians and virologists breathed a sigh of relief. But further outbreaks followed in Bangladesh and India in 2001, where they have since become a regular event, reminding us that while the battle may have been won, the war has not. Many researchers are now concerned that new strains of the virus might emerge in Africa, where contact with potential hosts such as bats and pigs is common.
Nipah can be contracted from bodily fluids of infected animals, and pigs are thought to catch the disease by eating fruit contaminated by infected bats or contact with bat droppings. Monitoring Nipah virus in livestock, as well as minimizing contact between humans and pigs, will be important measures to prevent a repeat jump of Nipah into humans from happening.
Currently Nipah virus can only be contracted by humans coming into contact with infected animals, although direct transmission from human to human has now been reported in a few cases. Since no antiviral treatment has been developed for the disease, the only way to slow an outbreak is to cull and carefully dispose of infected animals. There is no cure.
But it may be only a matter of time before Nipah–like HIV and Ebola before it–evolves the adaptations necessary to regularly jump from person to person. If it does, the next outbreak of Nipah may be more devastating than we can possibly imagine.

Read the next installment: “The Machine and the Mosquito“
Read the previous installment: “Outbreak: Our next global pandemic“
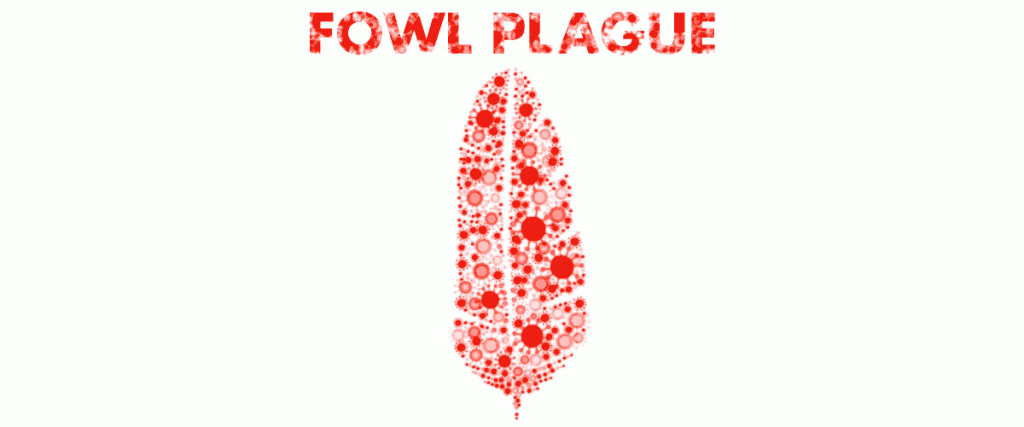
How We Get To Next was a magazine that explored the future of science, technology, and culture from 2014 to 2019. Fowl Plague is a five-part series that explores the history of deadly global pandemics–and asks whether we’re ready to respond to the next one.